Okataina Volcanic Centre Geology
By I.A. Nairn
Institute of Geological and Nuclear Sciences Ltd
Institute of Geological and Nuclear Sciences Ltd
INTRODUCTION

It is well known that Mt Tarawera is a volcano that erupted on 10 June 1886 AD, devastating the surrounding region and killing more than 100 of the sparse population living nearby at the time. Much less well known is that Mt Tarawera is only one volcano within the Okataina Volcanic Centre – an area of many recently active vents lying between Rotorua and Kawerau. These vents have erupted in prehistoric times, and will erupt again. The Okataina Centre includes the large young volcanoes of Tarawera and Haroharo, with others at Mt Edgecumbe, Okareka and Rotoma (figure 1). In contrast to some other New Zealand volcanoes such as Ngauruhoe and White Island, which have relatively small eruptions every few months or years, the volcanoes at Okataina have erupted at intervals which have varied between 700 and 3000 years. However, when eruptions do take place at Okataina, they are 100 to 10,000 times larger than those at White Island or Ngauruhoe. It is the size of most Okataina eruptions, despite their infrequency, that creates a significant volcanic hazard in the Bay of Plenty region. The risk from a volcanic eruption can be assessed from the relationship:
Probability of Eruption x Potential Damage = RISK
This indicates that although the risk from volcanic eruption at Okataina is not great, it cannot be ignored. Statistically, there is only about 1 chance in 20 that a large eruption will occur at Okataina in any 100 year period. However, when an eruption occurs it will be extremely damaging and probably affect much of the Bay of Plenty region between Tauranga, Opotiki, Murupara and Tokoroa.
Part of the 17 km long line of craters formed during the 1886 AD Tarawera-Rotomahana eruption within the Okataina Volcanic Centre. The 1886 craters extend from Waimangu in the foreground, across the very large craters now occupied by Lake Rotomahana, and on to Mt Tarawera beyond the lake. Many older volcanic vents are also shown in this photo, including Patiti Island in Lake Rotomahana, and Mt Edgecumbe in the far distance. Photo by D.L. Homer.
Probability of Eruption x Potential Damage = RISK
This indicates that although the risk from volcanic eruption at Okataina is not great, it cannot be ignored. Statistically, there is only about 1 chance in 20 that a large eruption will occur at Okataina in any 100 year period. However, when an eruption occurs it will be extremely damaging and probably affect much of the Bay of Plenty region between Tauranga, Opotiki, Murupara and Tokoroa.
Part of the 17 km long line of craters formed during the 1886 AD Tarawera-Rotomahana eruption within the Okataina Volcanic Centre. The 1886 craters extend from Waimangu in the foreground, across the very large craters now occupied by Lake Rotomahana, and on to Mt Tarawera beyond the lake. Many older volcanic vents are also shown in this photo, including Patiti Island in Lake Rotomahana, and Mt Edgecumbe in the far distance. Photo by D.L. Homer.
Figure 1: Map of the Okataina Volcanic Centre showing the margin of Haroharo Caldera (thick line) within which the ground surface has collapsed following enormous eruptions between 400,000 and 50,000 years ago. The caldera has since been partly infilled by the growth of young volcanoes at Tarawera, Haroharo, Rotoma and Ōkāreka during the last 20,000 years. Stars mark locations of vents active during this time.
|
ERUPTIONS TYPES

Although volcanic eruptions have occurred at Okataina for at least the last 400,000 years, most is known about the eruptions of the last 20,000 years. The eruptions during this time have been of several different types. Volcanic eruptions are classified firstly on the basis of the nature of material erupted, and secondly on the way in which the eruption occurs.
The volcanoes at Okataina have erupted two main types of magma (MAGMA is the molten rock underground prior to eruption). These magma types are BASALT and RHYOLITE. The distinction is based on chemistry, with the rhyolite containing more silica (see box) than does basalt. Rhyolite is often white of grey due to this higher silica content, while basalt is usually black or red. Pumice is rhyolite; scoria is basalt. The chemical distinction is important because it controls the viscosity (resistance to flow) of the erupting magma, and viscosity has an important influence on the style and violence of the eruption. Intermediate between basalts and rhyolites are ANDESITES and DACITES. These magmas have been erupted at Okataina only at Mt Edgecumbe and Waiotapu. Rhyolite eruptions are the most common at Okataina.
Volcanoes in the Okataina Centre are often not recognised as such. This photograph shows two rhyolite lava domes and a flow erupted from vents (asterisks) beyond the far shore of Lake Tikitapu (in foreground). The left-hand dome was formed during eruptions 21,000 years ago; the right-hand dome grew in the vent for the pumice deposits shown in Figure 8, which were erupted at 13,500 years ago. This eruption finished with extrusion of the thick rhyolite lava (grassed area) which extends from the vent down towards Lake Tarawera in middle distance. Mt Tarawera lies on the far shore of Lake Tarawera.
The volcanoes at Okataina have erupted two main types of magma (MAGMA is the molten rock underground prior to eruption). These magma types are BASALT and RHYOLITE. The distinction is based on chemistry, with the rhyolite containing more silica (see box) than does basalt. Rhyolite is often white of grey due to this higher silica content, while basalt is usually black or red. Pumice is rhyolite; scoria is basalt. The chemical distinction is important because it controls the viscosity (resistance to flow) of the erupting magma, and viscosity has an important influence on the style and violence of the eruption. Intermediate between basalts and rhyolites are ANDESITES and DACITES. These magmas have been erupted at Okataina only at Mt Edgecumbe and Waiotapu. Rhyolite eruptions are the most common at Okataina.
Volcanoes in the Okataina Centre are often not recognised as such. This photograph shows two rhyolite lava domes and a flow erupted from vents (asterisks) beyond the far shore of Lake Tikitapu (in foreground). The left-hand dome was formed during eruptions 21,000 years ago; the right-hand dome grew in the vent for the pumice deposits shown in Figure 8, which were erupted at 13,500 years ago. This eruption finished with extrusion of the thick rhyolite lava (grassed area) which extends from the vent down towards Lake Tarawera in middle distance. Mt Tarawera lies on the far shore of Lake Tarawera.
Magma
Basalt Andesite Dacite Rhyolite |
Silica Content
<53% 53-63% 63-68% >68% |
Viscosity
Low Medium Medium-High High |
Violence
Low to Moderate Moderate Low to High High |
Typical Eruption
Tarawera 1886 AD Ngauruhoe 1954 AD Mt St Helens 1980 AD Mt Tarawera 1200 AD |
Each type of magma can erupt in different ways. Explosive eruptions occur when expansion of the gas dissolved in the magma blows the magma apart as it reaches the ground surface. This fragmentation process has produced the basalt scoria and rhyolite ash and pumice which covers the entire area surrounding the Okataina Centre. These fragmented rocks are called PYROCLASTICS. If the magma can reach the surface without fragmenting, LAVA FLOWS are produced. Only rhyolite and dacite/andesite lave flows are found at Okataina, where they Okataina, where they typically pile up close to their vents to produce the steep sided cones of domes seen at Mt Edgecumbe (Figure 2), Tarawera and Haroharo.
Each type of eruption occurs when magma (of any type) encounters abundant groundwater (either hot or cold) at shallow depths. The magma, at 8000 to 11000C, heats the groundwater to generate large steam explosions which can be very violent. The 1886 AD explosions at Rotomahana were of this type, and caused nearly all the casualties resulting from the Tarawera eruption.
Each type of eruption occurs when magma (of any type) encounters abundant groundwater (either hot or cold) at shallow depths. The magma, at 8000 to 11000C, heats the groundwater to generate large steam explosions which can be very violent. The 1886 AD explosions at Rotomahana were of this type, and caused nearly all the casualties resulting from the Tarawera eruption.
Lava flows and domes from several vents have built up the complex Mt Edgecumbe dacite/andesite volcano at Kawerau within the last 7500 years. The youngest lavas of the summit cone are about 2500 years old. Large hydrothermal explosion craters are arrowed on the left side of the photo. These craters erupted at 15,000 and 9000 years ago. Kawerau pulp and paper mill is in the foreground. Photo by D.L. Homer.
|
OKATAINA ERUPTION HISTORY
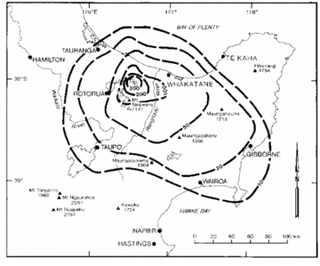
Between 400,000 and 50,000 years ago a series of five or six huge eruptions occurred at Okataina, removing about 500 km3 of magma from shallow depths in the earth’s crust, and causing the overlying ground surface to collapse and form an 18 km by 25 km basin, known as a CALDERA (see Figure 1). These huge eruptions devastated much of the Central North Island, burying large areas under ash and pumice 50m to 100m deep and so hot that the rock fragments welded together to form IGNIMBRITES. A series of eight smaller eruptions between 50,000 and 24,000 years ago also showered ash and pumice over much of the Central North Island, and modified the shape of the basin.
A detailed eruption history (Table 1) has been worked out for the last 21,000 years at Okataina. During this time more than 80 km3 of magma has been erupted in 11 distinct episodes, during which lavas and pyroclastic deposits have built up Haroharo, Tarawera and the other volcanoes. Each eruption episode was followed by a quiet period lasting for hundreds or thousands of years. Eruptions occurred from multiple vents during each episode, with craters up to 20 km apart in simultaneous or sequential activity. More than 40 vents have been active in the last 21,000 years, erupting mostly rhyolitic magma in pyroclastic explosions which have showered ash and pumice over large areas of the Bay of Plenty (Figure 4). The extrusion of thick rhyolite lava flows and domes has usually followed the explosive pyroclastic eruptions. Only relatively small volumes of basaltic magma have been erupted (Table 1). These were from Tarawera-Rotomahana area and also from a fissure that now contains the lakes Rotokawau-Rotoatua between lakes Rotoiti and Okataina (Figure 1).
Eruptions which built up the present Mt Tarawera first commenced about 18,000 years ago, producing the earliest pyroclastic deposits and lava flows which can be traced to this source. Other rhyolite eruption episodes at Tarawera occurred at 15,000, 11,000 and 800 years ago, before the 1886 AD basalt scoria eruption marked a dramatic change in the style of the volcano.
Mt Haroharo has a similar history, with the oldest pyroclastics and lavas being erupted at 21,000 years ago. Other Haroharo eruptions occurred at 9000, 7500 and 5000 years ago. Eruptions at Rotoma occurred about 9000 years ago, and at Ōkāreka at 21,000 and 13,500 years ago. Mt Edgecumbe has been built up within the last 7500 years, with most of the mountain formed within the last 5000 years. The most recent eruptions at Mt Edgecumbe occurred within the last 2500 years.
Figure 4: Map of the present day distribution and thickness of the pyroclastic fall deposit erupted from the Okataina Centre 9000 years ago. The dashed lines enclose areas in which the deposit thickness exceeds the value (in centimetres) shown on the line. Erosion and compaction of the deposit since eruption means that the original thickness may have been up to twice the present day thickness.
A detailed eruption history (Table 1) has been worked out for the last 21,000 years at Okataina. During this time more than 80 km3 of magma has been erupted in 11 distinct episodes, during which lavas and pyroclastic deposits have built up Haroharo, Tarawera and the other volcanoes. Each eruption episode was followed by a quiet period lasting for hundreds or thousands of years. Eruptions occurred from multiple vents during each episode, with craters up to 20 km apart in simultaneous or sequential activity. More than 40 vents have been active in the last 21,000 years, erupting mostly rhyolitic magma in pyroclastic explosions which have showered ash and pumice over large areas of the Bay of Plenty (Figure 4). The extrusion of thick rhyolite lava flows and domes has usually followed the explosive pyroclastic eruptions. Only relatively small volumes of basaltic magma have been erupted (Table 1). These were from Tarawera-Rotomahana area and also from a fissure that now contains the lakes Rotokawau-Rotoatua between lakes Rotoiti and Okataina (Figure 1).
Eruptions which built up the present Mt Tarawera first commenced about 18,000 years ago, producing the earliest pyroclastic deposits and lava flows which can be traced to this source. Other rhyolite eruption episodes at Tarawera occurred at 15,000, 11,000 and 800 years ago, before the 1886 AD basalt scoria eruption marked a dramatic change in the style of the volcano.
Mt Haroharo has a similar history, with the oldest pyroclastics and lavas being erupted at 21,000 years ago. Other Haroharo eruptions occurred at 9000, 7500 and 5000 years ago. Eruptions at Rotoma occurred about 9000 years ago, and at Ōkāreka at 21,000 and 13,500 years ago. Mt Edgecumbe has been built up within the last 7500 years, with most of the mountain formed within the last 5000 years. The most recent eruptions at Mt Edgecumbe occurred within the last 2500 years.
Figure 4: Map of the present day distribution and thickness of the pyroclastic fall deposit erupted from the Okataina Centre 9000 years ago. The dashed lines enclose areas in which the deposit thickness exceeds the value (in centimetres) shown on the line. Erosion and compaction of the deposit since eruption means that the original thickness may have been up to twice the present day thickness.
Volcano in Eruption
Tarawera/Rotomahana
Tarawera Rotokawau Haroharo Haroharo Rotoma/Haroharo Tarawera Ōkāreka Tarawera Tarawera Haroharo/Okareka |
Magma Volume (km3)
1
5 0.5 13 17 8 10 4 5 7 9 |
Eruption Type
B
R B R R R R R R B/R R |
Age
(Years Ago) 1886 AD
800 3500 5000 7500 9000 11,000 13,500 15,000 18,000(?) 21,000 |
Quiescence (Years)
700
2700 1500 2500 1500 2000 2500 1500 3000 3000? 3000 |
Table 1: Magma volumes, types, ages and quiescent intervals between eruptions at Okataina Volcanic Centre during the past 21,000 years. B = basalt, R = rhyolite
THE 1886 AD TARAWERA RIFT ERUPTION

The 1886 eruption is the largest to have occurred in New Zealand’s recorded history. There had been no signs of impending activity, such as hot spring of steam emission, on Mt Tarawera prior to June 1886. On 1 June, peculiar waves 0.3m high on the western shore of Lake Tarawera may have resulted from early ground movements, but the first definite indications of impending eruption were earthquakes felt at Te Wairoa and Rotorua beginning at 12.30am on 10 June. These earthquakes increased in intensity until the eruption began about one hour later. By 2.30am craters along the full length of the mountain were in eruption, producing ash column more than 10 km high. Rotomahana apparently started erupting at about 3.20 am, when a severe earthquake occurred. A high eruption column which rose at 2.30am from a vent further southwest, probably from Waimangu, apparently preceded the main Rotomahana explosions. Eruptions continued from the entire 17 km long rift until about 6.00am, showering basalt scoria and mud across a large area of the Bay of Plenty with ash also falling on ships off the east coast, 220 km from the source.
The eruptions at Rotomahana were particularly explosive, as the basalt magma reacted with the existing intense hydrothermal system. Steam explosions blew out large craters, and formed an 11 km high eruption column. The explosive expansion of superheated water fragmented the rock containing the hydrothermal system to produce the "Rotomahana Mud" which fell over a wide area of the Bay of Plenty. Near the source, ground-hugging turbulent hurricanes ("surges") of mud, ash and steam swept up over the summits of hills standing 360m above the Rotomahana crater floor, and travelled more than 6 km to the west. The site of the Maori village at Te Ariki was overwhelmed by surges, while an island camp in the middle of the pre-eruption Lake Rotomahana was simply blown away. Buildings at Te Wairoa collapsed under the mudfall (see Inside Back Cover). Most of the more than 100 eruption fatalities resulted from explosions at Rotomahana, rather than at Mt Tarawera.
Spectacular lightning occurred in the eruption cloud, and fireballs or lightning set fire to a house at Te Wairoa and to the forest on the north shore of Lake Tarawera. Fissures opened on the numerous faults to the southwest of Waimangu, making travel across country difficult. Strong winds during the eruption blew over many trees at Tikitapu (Blue Lake), while suffocating gases and falling ash made breathing difficult at Te Wairoa. The northward passage of the eruption cloud caused darkness during daylight hours at Rotorua, Te Puke, Tauranga, Whakatane, Opotiki and East Cape.
The 1886 AD Tarawera Basalt deposits are well exposed along the eruption rift formed across Mt Tarawera where the red and black scoria varies in thickness from 20-60m. The basalt contrasts with the older grey and white rhyolite lavas and pyroclastics which make up the great bulk of Mt Tarawera. Weak steam vents in the rift craters are now the only indication of subterranean heat on Mt Tarawera.
Although the 1886 Tarawera eruption is the largest to have occurred in New Zealand during the last 200 years, it is important to realise that this was one of the smallest eruptions at Okataina during the last 21,000 years. The next eruption at Okataina is expected to be similar to those of the last 21,000 years, i.e. it is very likely to be larger and more damaging than the 1886 event.
Figure 6: Some of the craters formed on the summit of Mt Tarawera during the 1886 AD eruption. The dark basaltic scoria fall overlies the white rhyolite pumice and lavas (grey) of the 800 year Tarawera eruption. Photo by D.L. Homer.
The eruptions at Rotomahana were particularly explosive, as the basalt magma reacted with the existing intense hydrothermal system. Steam explosions blew out large craters, and formed an 11 km high eruption column. The explosive expansion of superheated water fragmented the rock containing the hydrothermal system to produce the "Rotomahana Mud" which fell over a wide area of the Bay of Plenty. Near the source, ground-hugging turbulent hurricanes ("surges") of mud, ash and steam swept up over the summits of hills standing 360m above the Rotomahana crater floor, and travelled more than 6 km to the west. The site of the Maori village at Te Ariki was overwhelmed by surges, while an island camp in the middle of the pre-eruption Lake Rotomahana was simply blown away. Buildings at Te Wairoa collapsed under the mudfall (see Inside Back Cover). Most of the more than 100 eruption fatalities resulted from explosions at Rotomahana, rather than at Mt Tarawera.
Spectacular lightning occurred in the eruption cloud, and fireballs or lightning set fire to a house at Te Wairoa and to the forest on the north shore of Lake Tarawera. Fissures opened on the numerous faults to the southwest of Waimangu, making travel across country difficult. Strong winds during the eruption blew over many trees at Tikitapu (Blue Lake), while suffocating gases and falling ash made breathing difficult at Te Wairoa. The northward passage of the eruption cloud caused darkness during daylight hours at Rotorua, Te Puke, Tauranga, Whakatane, Opotiki and East Cape.
The 1886 AD Tarawera Basalt deposits are well exposed along the eruption rift formed across Mt Tarawera where the red and black scoria varies in thickness from 20-60m. The basalt contrasts with the older grey and white rhyolite lavas and pyroclastics which make up the great bulk of Mt Tarawera. Weak steam vents in the rift craters are now the only indication of subterranean heat on Mt Tarawera.
Although the 1886 Tarawera eruption is the largest to have occurred in New Zealand during the last 200 years, it is important to realise that this was one of the smallest eruptions at Okataina during the last 21,000 years. The next eruption at Okataina is expected to be similar to those of the last 21,000 years, i.e. it is very likely to be larger and more damaging than the 1886 event.
Figure 6: Some of the craters formed on the summit of Mt Tarawera during the 1886 AD eruption. The dark basaltic scoria fall overlies the white rhyolite pumice and lavas (grey) of the 800 year Tarawera eruption. Photo by D.L. Homer.
PRODUCTS OF ERUPTIONS Pyroclastic Fall Deposits (Tephra)
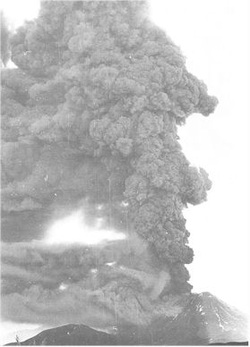
During an explosive rhyolite eruption the magma is torn apart by the expansion of dissolved gases (an effect rather like shaking an open bottle of fizzy drink) and the resulting particles of solidifying magma are carried by the escaping gas up into the air as ash and pumice. The eruption column above the volcano (Figure 7), driven by expansion of heated air, rises by convection to heights of 20-30 km for typical Okataina rhyolite eruptions, and expands into an eruption cloud which drifts downwind. Ash and pumice falls out of the cooling cloud, the larger particles falling closest to the vent where the PYROCLASTIC FALL (or TEPHRA) deposit is thickest (Figure 8), with fine volcanic ash drifting up to hundreds or thousands of kilometres from the vent, as the deposit gradually thins. A map of the distribution and thickness of a typical pryoclastic fall deposit from Okataina is shown in Figure 4.
Close to the eruption vent, the fall deposits can be of damaging thickness (more than 0.5m) causing collapse and burning of buildings, burial of animals and people, and destruction of vegetation. The deaths at Te Wairoa (Buried Village) during the 1886 AD eruption were due to pyroclastic fall. However, more distant areas were covered by thinner deposits, which although survivable, caused considerable disruption to normal activities. Pyroclastic falls can cause total darkness during daytime, affect breathing (particularly for people with respiratory problems), contaminate water supplies, and damage machinery such as car and aircraft motors. Electrical storms are usually associated with large pyroclastic eruptions. Lightning strikes, together with wet ash on insulators and antennas, can disrupt radio and telephone communications and electricity reticulation. Pyroclastic falls damage pastures in the short term, but can improve fertility in the long term (all Bay of Plenty region soils are developed on pyroclastic deposits). Volcanic ash can affect farm animals by burial of pasture, wear on teeth, and effects on digestive systems. Some pyroclastic falls overseas have been poisonous because of the presence of fluorine aerosols or other toxic compounds, but ash from future eruptions of Okataina is not expected to be toxic.
Even this ash fall can immobilise vehicles with engine failures or blocked air filters. Passage of vehicles stirs up clouds of fine ash from roads, even after ash has stopped falling.
Figure 7: An eruption column at Ngauruhoe in 1974, rising to about 5 km above the volcano. The rhyolite eruptions Okataina have produced columns which rose 5 – 10 times higher than this Ngauruhoe (andesite) example, and deposited 1000 – 10,000 times more ash. Photo by J. Scobie
Close to the eruption vent, the fall deposits can be of damaging thickness (more than 0.5m) causing collapse and burning of buildings, burial of animals and people, and destruction of vegetation. The deaths at Te Wairoa (Buried Village) during the 1886 AD eruption were due to pyroclastic fall. However, more distant areas were covered by thinner deposits, which although survivable, caused considerable disruption to normal activities. Pyroclastic falls can cause total darkness during daytime, affect breathing (particularly for people with respiratory problems), contaminate water supplies, and damage machinery such as car and aircraft motors. Electrical storms are usually associated with large pyroclastic eruptions. Lightning strikes, together with wet ash on insulators and antennas, can disrupt radio and telephone communications and electricity reticulation. Pyroclastic falls damage pastures in the short term, but can improve fertility in the long term (all Bay of Plenty region soils are developed on pyroclastic deposits). Volcanic ash can affect farm animals by burial of pasture, wear on teeth, and effects on digestive systems. Some pyroclastic falls overseas have been poisonous because of the presence of fluorine aerosols or other toxic compounds, but ash from future eruptions of Okataina is not expected to be toxic.
Even this ash fall can immobilise vehicles with engine failures or blocked air filters. Passage of vehicles stirs up clouds of fine ash from roads, even after ash has stopped falling.
Figure 7: An eruption column at Ngauruhoe in 1974, rising to about 5 km above the volcano. The rhyolite eruptions Okataina have produced columns which rose 5 – 10 times higher than this Ngauruhoe (andesite) example, and deposited 1000 – 10,000 times more ash. Photo by J. Scobie
Pyroclastic Flows
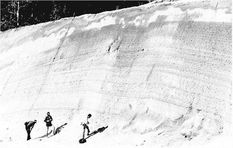
A 20 – 30 km high eruption column composed of hot gas, ash and pumice particles comprises an enormous mass of material poised high above the surrounding countryside. The column stays up only because the hot gas it contains maintains a column density lower than that of the surrounding atmosphere. If the column becomes denser than the atmosphere it is no longer buoyant, and collapses with an effect rather like turning on the tap into a kitchen sink, magnified 100,000 times. The column can fall out of the sky at great speed, and on reaching the ground, this downward velocity is transformed into horizontal speed over the ground surface, forming a PYROCLASTIC FLOW or SURGE. A radially expanding flood of hot ash and pumice fragments in a turbulent gas cloud flows outwards over the ground surface at speeds which can exceed 200 kilometres an hour. Pyroclastic flows and surges can also form in other ways, but the effects are the same – the total and almost instantaneous devastation of the entire area covered by the flow. Unlike lava flows, it is not possible to outrun a pyroclastic flow, and their high velocities and hot temperatures make them especially dangerous.
Nearly all the Okataina eruptions of the last 21,000 years have generated pyroclastic flows and surges near their vents, and these are the most destructive manifestations of volcanic activity. Most of the deaths in the 1886 AD Tarawera eruption were caused by pyroclastic surges from the Rotomahana area.
A particular type of pyroclastic flow is the hot avalanche caused by the collapse of part of a growing lava dome. Hot lava boulders, ash and gas cascade down the side of a volcano. The best examples at Okataina are found around Mt Edgecumbe, and also Mt Tarawera where they occurred after the main ash-producing eruptions. The hot avalanches at Mt Tarawera were produced by collapse of the steep margins of the summit domes during the last rhyolite eruption 800 years ago, and extend out to 10 km from the volcano.
Figure 8: Pyroclastic fall ash and pumice deposits erupted from vents between lakes Okareka and Tikitapu at 13,500 years ago. The entire deposit exposed in this road cutting was probably erupted over a few days. The road cutting is 4 km from the source vent, which is shown on the Inside Front Cover.
Nearly all the Okataina eruptions of the last 21,000 years have generated pyroclastic flows and surges near their vents, and these are the most destructive manifestations of volcanic activity. Most of the deaths in the 1886 AD Tarawera eruption were caused by pyroclastic surges from the Rotomahana area.
A particular type of pyroclastic flow is the hot avalanche caused by the collapse of part of a growing lava dome. Hot lava boulders, ash and gas cascade down the side of a volcano. The best examples at Okataina are found around Mt Edgecumbe, and also Mt Tarawera where they occurred after the main ash-producing eruptions. The hot avalanches at Mt Tarawera were produced by collapse of the steep margins of the summit domes during the last rhyolite eruption 800 years ago, and extend out to 10 km from the volcano.
Figure 8: Pyroclastic fall ash and pumice deposits erupted from vents between lakes Okareka and Tikitapu at 13,500 years ago. The entire deposit exposed in this road cutting was probably erupted over a few days. The road cutting is 4 km from the source vent, which is shown on the Inside Front Cover.
Lava Flows and Domes

Lava flows and domes are formed when magma which has lost most of its dissolved gas is erupted. Often this occurs after the explosive eruption of ash has discharged most of the "frothy" top to the underground magma body. Rhyolite lava flows are so viscous that they move very slowly, and do not generally threaten lives, but they destroy any structures in their paths, and can start forest fires. At Okataina, lava flows have usually only buried areas already devastated by pyroclastic flows and thick ash falls, but when they have flowed into the outlets of lakes they have caused widespread flooding (see below).
Steep-sided, often circular rhyolite domes form when the rhyolite magma is so viscous that it cannot flow far, but instead piles up around the vent. The three 800 year old summit domes of Mt Tarawera were typical of this type of extrusion (Figure 9). As with lava flows, growth of domes poses little direct threat to human life except from the generation of hot avalanches.
Figure 9: The three lava domes forming the summit of Mt Tarawera were extruded about 800 years ago and overlie older lava and pyroclastic deposits. Photo by D.L. Homer.
Steep-sided, often circular rhyolite domes form when the rhyolite magma is so viscous that it cannot flow far, but instead piles up around the vent. The three 800 year old summit domes of Mt Tarawera were typical of this type of extrusion (Figure 9). As with lava flows, growth of domes poses little direct threat to human life except from the generation of hot avalanches.
Figure 9: The three lava domes forming the summit of Mt Tarawera were extruded about 800 years ago and overlie older lava and pyroclastic deposits. Photo by D.L. Homer.
Hydrothermal Eruptions
Another type of eruption that has occurred at Okataina is a steam explosion in hydrothermal fields. Steam explosions occurred at Rotomahana in 1886 AD, Waimangu in 1915 and 1917 AD, and at Waiotapu, Kawerau, Rotoma and Tikitere in prehistoric times. In boiling hydrothermal systems, the hot water at depth can flash into steam if the confining pressure is reduced, to produce powerful explosions which eject hot rocks and mud, hot water and steam. Confining pressures on hydrothermal systems can be reduced by fault fracturing during earthquakes, and by the fracturing associated with the intrusion of magma at nearby or distant volcanic eruption sites. The 1886 AD Rotomahana hydrothermal eruptions were triggered by fracturing associated with basalt magma intrusion into the pre-existing hydrothermal system, and occurred simultaneously with the magmatic eruptions from Mt Tarawera. Hydrothermal eruptions at Waiotapu occurred during the last rhyolite eruption from Tarawera about 800 years ago. Other large hydrothermal eruptions at Kawerau appear to have closely followed distant volcanic eruptions at Rotoma 9000 years ago and at Tarawera 15,000 years ago, and were probably triggered by them. However, the hydrothermal eruptions which occurred at Tikitere do not appear to have been related to any volcanic eruption, but possibly to changes in the levels of the nearby lakes Rotoiti and Rotorua, which affected groundwater levels and hence the confining pressures on the hydrothermal system.
Damage from hydrothermal eruptions usually extends out to a few hundred metres from the vent i.e. they are usually smaller than magmatic eruptions.
Damage from hydrothermal eruptions usually extends out to a few hundred metres from the vent i.e. they are usually smaller than magmatic eruptions.
Volcanic Gases
Volcanic eruptions are driven by expanding gases, principally water vapour and carbon dioxide (CO2), but including poisonous gases such as sulphur dioxide (SO2) and hydrogen sulphide (H2S). Only very close to vents are these gases present in toxic amounts, but at greater distances they often become dissolved in atmospheric clouds to produce acid rains and mists which affect human and animal eyes and respiratory systems, as well as damaging vegetation and metal building materials. Surface water supplies can also become acidified. Gas masks provide personal protection against poisonous gases, and masks can be substituted for by breathing through moistened cloths in an emergency. However, CO2 and H2S are both denser than air and can accumulate in depressions and closed buildings, where they can cause death by asphyxiation, even if gas masks are worn. Volcanic gases continue to be released after eruptions have ceased, so that a gas hazard can continue long after an eruption hazard.
Forest Fires
Exotic pine forests now cover much of the Okataina Volcanic Centre and extend for great distances to the north and south of the centre. During dry periods in late summer, a high to extreme fire hazard is reported in these forests. If a future eruption were to occur during a period of high fire risk, forests could be set alight by hot pyroclastic material, lava flows, or lightning strikes. The spread of any fire would then depend on wind directions and the locations of clear-felled areas and natural barriers such as lakes. Large areas of forest could be burnt, causing hazard to life in areas perhaps not directly affected by the eruption.
Lahars and Floods
Each eruption at Tarawera and Haroharo during the last 10,000 years has been followed by LAHARS down the Tarawera River and other streams. A lahar is a dense mudflow, rather similar to wet concrete, formed when volcanic material is mixed with water. At Okataina the lahars seem to have occurred when dams of pyroclastic debris, formed after each eruption, were overtopped or collapsed to precipitate a flood of debris down the Tarawera River channel to beyond Kawerau. The river terraces on which much of Kawerau is built were formed by these lahars. Because some time is required to collect water behind the eruption-formed dam, the largest lahars must have occurred months or years after their associated eruptions. For example, the outlet to Lake Tarawera was blocked during the 1886 AD eruption and the lake rose to 13m above its pre-eruption level. The dam was overtopped and largely carried away in November 1904 AD when a lahar flowed down the Tarawera River and flooded the Kawerau area.
Although most lahars at Okataina have occurred after the eruption has ended, there is also a slight possibility of immediate catastrophic flooding of the Tarawera River if explosive eruptions were to remove narrow land barriers separating Lake Tarawera from the adjoining lakes, all of which are at higher elevations (Figure 3). Explosion craters exist in lakes Rotomahana and Rerewhakaaitu, and a 600m diameter explosion crater lies between lakes Okataina, Ōkāreka and Tarawera. Although the probability of an explosion crater forming in exactly the right location to breach a divide between lakes is very low, the risk of catastrophic flooding of the Kawerau area during any future eruption at Okataina should be recognised. Lake Rotoma also contains 9000 year old explosion craters near its western end, and any recurrence of explosive activity here could remove the narrow barrier which confines the western are of the lake, allowing flooding into the Rotoehu area.
Large waves are likely to be generated by the rapid entry of pyroclastic flows and debris avalanches into lakes during a future eruption at the Okataina Centre. Volcanic explosions occurring within lakes (e.g. form the existing vents at Rotoma and Rotomahana) would also produce locally-damaging large waves. If volumes of displaced water are large in lakes Rotoiti and Tarawera, floods could pass down the Kaituna and Tarawera Rivers, respectively.
Lava flows can also cause long-term flooding of lake shores if they block the outlets of overflowing lakes. For example, Lake Tarawera rose more than 30m to about its present level following a lava flow into the outlet area about 5000 years ago. Such long-term level changes pose no threat to human life but would submerge roads and buildings around lake shores.
Although most lahars at Okataina have occurred after the eruption has ended, there is also a slight possibility of immediate catastrophic flooding of the Tarawera River if explosive eruptions were to remove narrow land barriers separating Lake Tarawera from the adjoining lakes, all of which are at higher elevations (Figure 3). Explosion craters exist in lakes Rotomahana and Rerewhakaaitu, and a 600m diameter explosion crater lies between lakes Okataina, Ōkāreka and Tarawera. Although the probability of an explosion crater forming in exactly the right location to breach a divide between lakes is very low, the risk of catastrophic flooding of the Kawerau area during any future eruption at Okataina should be recognised. Lake Rotoma also contains 9000 year old explosion craters near its western end, and any recurrence of explosive activity here could remove the narrow barrier which confines the western are of the lake, allowing flooding into the Rotoehu area.
Large waves are likely to be generated by the rapid entry of pyroclastic flows and debris avalanches into lakes during a future eruption at the Okataina Centre. Volcanic explosions occurring within lakes (e.g. form the existing vents at Rotoma and Rotomahana) would also produce locally-damaging large waves. If volumes of displaced water are large in lakes Rotoiti and Tarawera, floods could pass down the Kaituna and Tarawera Rivers, respectively.
Lava flows can also cause long-term flooding of lake shores if they block the outlets of overflowing lakes. For example, Lake Tarawera rose more than 30m to about its present level following a lava flow into the outlet area about 5000 years ago. Such long-term level changes pose no threat to human life but would submerge roads and buildings around lake shores.
Volcanic Earthquakes and Faulting
Earthquakes will almost certainly precede and accompany any volcanic eruption at Okataina. Although volcanic earthquakes rarely exceed magnitude 5 (i.e. they are not particularly large in terms of total energy release) their shallow depths and proximity to population centres at Okataina means they will be strongly felt. Such earthquakes may cause damage in areas that may not be directly affected by the eruption. For example, the earthquakes which accompanied the 1886 AD Tarawera eruption caused considerable ground cracking and fissuring on the many faults which cross the Waiotapu-Waimangu area to the southwest of the Okataina Centre, so that it was difficult to take a horse across country. Road access in this and other areas of intense faulting, i.e. to the north of Rotoma, may become blocked by faulting or fissuring in any future eruption.
HAZARD ZONES

The next eruption at Okataina is expected to resemble those which have occurred during the last 21,000 years, rather than the much larger eruptions of earlier times.
The areas at risk during a future eruption from the Okataina Centre will depend on location of the active vents, local topography in the vent areas, wind directions at the time of the eruption, and the magnitude, style and duration of the eruption. The variety of eruptions which have occurred during the past 21,000 years makes it difficult to predict any of these factors for the next eruption. Until likely vent locations and style of an impending eruption can be more precisely defined by precursory activity, hazard zoning at Okataina can only be indicated in broad terms (Figure 10, 11).
A rhyolite eruption will most probably occur on one of the two vent zones that pass through the Haroharo and Tarawera volcanoes (see Figures 1, 3), with multiple vents located anywhere along the active vent zone. For example, vents active during a Haroharo eruption could be located anywhere between Lake Rotoma and Lake Tikitapu. The areas surrounding the erupting vents will be completely devastated by pyroclastic flows and surges. These areas will partly controlled by local topography, and extend out to about 10 km from the vents.
Figure 10: Hazard zones defined at Okataina Volcanic Centre based on the effects of past eruptions. The hatching defines areas at risk of complete devastation as a result of eruptions at (A) Haroharo; (B) Tarawera; (C) Mt Edgecumbe. The dashed lines enclose areas at risk from hydrothermal eruptions. The dot pattern defines rivers at risk of eruption-induced flooding and lahars. The risk is much greater for the Tarawera River than for the Kaituna River.
Areas at risk of deep burial (greater than 0.5m) by pyroclastic fall ejecta (tephra) will lie downwind of the vent locations. With prevailing westerly winds (Figure 11) the thickest deposits are most likely to be dispersed to the east, where many previously erupted deposits are thickest. However, eruptions may occur during winds from less common directions, and thick pyroclastic fall deposits could accumulate in any sector. A previous example is the 13,500 year old Rotorua Ash with its dispersal axis to the northwest from its source near Lake Tikitapu. A similar eruption today would largely bury Rotorua City beneath about 2m ash and pumice.
The extrusion of lava flows and domes will probably follow rhyolite pyroclastic eruptions. Areas at risk of burial by lavas are probably smaller than those shown for pyroclastic flows, and would be largely confined within Haroharo Caldera.
A basalt eruption is most likely to recur from fissure vents at Tarawera-Rotomahana, but could also occur at apparently random locations within or outside Haroharo Caldera. A future basalt eruption will most probably produce scoria falls, affecting smaller areas than the typical rhyolite pyroclastic eruptions. If basalt eruptions occur in low-lying areas they are likely to be highly explosive events, producing relatively small pyroclastic surges. Near-vent areas of complete devastation will probably extend out to about 5 km (i.e. less than for rhyolite eruptions). As with the rhyolitic deposits, areas of deep burial will largely depend on wind direction at the time of the eruption. Because a basalt eruption will probably be of shorter duration than a rhyolite one, with lower eruption columns, dispersal directions should be more tightly confined. This is because there will be less opportunity for changes in wind directions during the eruption, and/or the eruption column to penetrate overlying layers of the atmosphere in which wind directions may be different.
A dacitic/andesitic eruption may occur at Mt Edgecumbe, with a lesser possibility of eruption at Waiotapu, where Maungakakaramea (Rainbow Mountain) and Maungaongaonga are older dacitic volcanoes. No widespread pyroclastic deposits are associated with any of these dacitic volcanoes, suggesting that a future eruption is also likely to directly affect only their immediate areas. The main risks at Mt Edgecumbe and Waiotapu would come from hot pyroclastic flows and avalanches sweeping down form summit vents, and from hydrothermal eruptions which might be triggered in the adjacent hydrothermal fields. However, Mt Edgecumbe is such a young and rapidly constructed volcano that any future eruptions might be quite different from those of the past. An explosive dome collapse leading to pyroclastic surges/flows and hot avalanches would have to be considered possible in any future activity at this volcano. Lava flows from the multiple vents at Mt Edgecumbe might extend into the Tarawera River, damming and/or diverting the river west towards the Kawerau township.
The areas at risk during a future eruption from the Okataina Centre will depend on location of the active vents, local topography in the vent areas, wind directions at the time of the eruption, and the magnitude, style and duration of the eruption. The variety of eruptions which have occurred during the past 21,000 years makes it difficult to predict any of these factors for the next eruption. Until likely vent locations and style of an impending eruption can be more precisely defined by precursory activity, hazard zoning at Okataina can only be indicated in broad terms (Figure 10, 11).
A rhyolite eruption will most probably occur on one of the two vent zones that pass through the Haroharo and Tarawera volcanoes (see Figures 1, 3), with multiple vents located anywhere along the active vent zone. For example, vents active during a Haroharo eruption could be located anywhere between Lake Rotoma and Lake Tikitapu. The areas surrounding the erupting vents will be completely devastated by pyroclastic flows and surges. These areas will partly controlled by local topography, and extend out to about 10 km from the vents.
Figure 10: Hazard zones defined at Okataina Volcanic Centre based on the effects of past eruptions. The hatching defines areas at risk of complete devastation as a result of eruptions at (A) Haroharo; (B) Tarawera; (C) Mt Edgecumbe. The dashed lines enclose areas at risk from hydrothermal eruptions. The dot pattern defines rivers at risk of eruption-induced flooding and lahars. The risk is much greater for the Tarawera River than for the Kaituna River.
Areas at risk of deep burial (greater than 0.5m) by pyroclastic fall ejecta (tephra) will lie downwind of the vent locations. With prevailing westerly winds (Figure 11) the thickest deposits are most likely to be dispersed to the east, where many previously erupted deposits are thickest. However, eruptions may occur during winds from less common directions, and thick pyroclastic fall deposits could accumulate in any sector. A previous example is the 13,500 year old Rotorua Ash with its dispersal axis to the northwest from its source near Lake Tikitapu. A similar eruption today would largely bury Rotorua City beneath about 2m ash and pumice.
The extrusion of lava flows and domes will probably follow rhyolite pyroclastic eruptions. Areas at risk of burial by lavas are probably smaller than those shown for pyroclastic flows, and would be largely confined within Haroharo Caldera.
A basalt eruption is most likely to recur from fissure vents at Tarawera-Rotomahana, but could also occur at apparently random locations within or outside Haroharo Caldera. A future basalt eruption will most probably produce scoria falls, affecting smaller areas than the typical rhyolite pyroclastic eruptions. If basalt eruptions occur in low-lying areas they are likely to be highly explosive events, producing relatively small pyroclastic surges. Near-vent areas of complete devastation will probably extend out to about 5 km (i.e. less than for rhyolite eruptions). As with the rhyolitic deposits, areas of deep burial will largely depend on wind direction at the time of the eruption. Because a basalt eruption will probably be of shorter duration than a rhyolite one, with lower eruption columns, dispersal directions should be more tightly confined. This is because there will be less opportunity for changes in wind directions during the eruption, and/or the eruption column to penetrate overlying layers of the atmosphere in which wind directions may be different.
A dacitic/andesitic eruption may occur at Mt Edgecumbe, with a lesser possibility of eruption at Waiotapu, where Maungakakaramea (Rainbow Mountain) and Maungaongaonga are older dacitic volcanoes. No widespread pyroclastic deposits are associated with any of these dacitic volcanoes, suggesting that a future eruption is also likely to directly affect only their immediate areas. The main risks at Mt Edgecumbe and Waiotapu would come from hot pyroclastic flows and avalanches sweeping down form summit vents, and from hydrothermal eruptions which might be triggered in the adjacent hydrothermal fields. However, Mt Edgecumbe is such a young and rapidly constructed volcano that any future eruptions might be quite different from those of the past. An explosive dome collapse leading to pyroclastic surges/flows and hot avalanches would have to be considered possible in any future activity at this volcano. Lava flows from the multiple vents at Mt Edgecumbe might extend into the Tarawera River, damming and/or diverting the river west towards the Kawerau township.
Figure 11: A map of the possible distribution of a future rhyolite pyroclastic fall deposit from the Okataina Centre, based on past eruption deposits. The entire area shown may be covered by deposits less than 0.1m thick, but direction of ash dispersal will be controlled by wind directions during the eruption. The compass diagram shows the percentages of time that winds blow towards various sectors, i.e. winds blow from west to east 40% of the time. It is possible, but much less likely, that an eruption could occur during winds blowing in the opposite direction (about 2% of the time).
|
Hydrothermal explosions in hydrothermal fields associated with the Okataina Centre may be triggered by a volcanic eruption or major earthquake. Although locally devastating, the significant effects of hydrothermal eruptions are unlikely to extend more than a few kilometres from the source vents (Figure 10). Rotomahana provides the main exception to this statement. The combination of a large hydrothermal system, abundant lake water, and fissure sites of repeated past basaltic and rhyolitic eruptions indicates that large steam eruptions will recur at Rotomahana, and may affect areas as large as those of the 1886 AD eruption.
The Tarawera River and its floodplain (Figure 10) are at some risk of flooding during and following eruptions at both Tarawera and Haroharo. The flood hazard during eruptions arises from the slight possibility of explosive breaching of the divides separating lakes. Floods generated at such a time could occur with little or no warning. Other floods which will follow the filling and overtopping or breaching of pyroclastic dams should be anticipated, and suitable precautionary measures taken. Some flood risk also exists at Lake Rotoehu, and possibly at Lake Rotoiti and down the Kaituna River, if explosive eruptions at Lake Rotoma were to breach the western margin of the lake. Large waves would also affect the margins of the lakes of the Okataina Centre if large pyroclastic flows or avalanches rapidly displace considerable quantities of lake water, or if volcanic explosions occur at vents in the lakes. |
EVENTS LEADING TO AN ERUPTION

The course of events likely to precede the next eruption at Okataina depends largely on whether the eruption is of basaltic or rhyolitic type. If the next eruption is basaltic it will probably occur with only a short period of seismicity, detected over a few days, or at most weeks, before the eruption. (The 1886 AD Tarawera eruption was preceded by no more than 1 hour of felt earthquakes!) This is because a basaltic eruption will probably involve a relatively small volume (» 1 km3) of low-viscosity magma which will rapidly fracture its way up through the earth’s crust from a depth of about 15 km. It will be difficult to distinguish the seismicity associated with this fracturing from the frequent non-volcanic earthquake swarms which now occur without associated eruptions, about once per year in the Okataina Centre.
Deformation of the ground surface above the earthquake locations will probably precede and would help identify an impending eruption, if the deformation occurs within previously surveyed networks, such as those installed at Tarawera-Rotomahana, or near lakes used as tilt-level indicators, so that it can be instrumentally measured (see Figure 12). Ground deformation occurring elsewhere may not be detectable until becoming visible to the naked eye, probably immediately prior to the eruption.
The short duration anticipated for precursory seismicity and ground deformation suggests that a basaltic eruption may not be usefully predicted. The eruption may occur before it could be confidently recognised as definitely impending.
In contrast, a rhyolite eruption is likely to be preceded by a long period (months or years) of increased shallow seismicity, with noticeable ground deformation occurring above the potential vent areas, changes in lake levels, and possibly increased heat flows from hot springs and fumarolic (steaming) areas. This is because rhyolitic eruptions are thought to come from large (10-100 km3?) viscous magma bodies stored at shallow depths (5-10 km?) in the earth’s crust. Magma intrusions rising from these large bodies will slowly fracture their way towards the surface.
Unlike basaltic eruptions, precursory indications of an impending rhyolitic eruption are likely to be recognised long before the event. However, while all large rhyolite eruptions are likely to be predicted, these predictions may include many false alarms. During the 1980s, active calderas in Italy, the United States and Papua New Gineau, went through periods of considerable unrest. Levels of seismic activity increased greatly, and vertical ground deformation (swelling) of 1m or larger was measured. These changes, although alarming and best explained by underground movement of magma, have not (as yet) led to eruptions.* It appears that calderas may undergo periods of considerable disturbance more frequently than actual eruptions may occur. There is no immediate one-to-one correlation between unrest and eruption. Thus, if a period of unrest occurs at Okataina, with greatly increased seismicity and ground deformation, it will indicate an increased probability, rather than a certainty, of impending eruption.
*Except at Rabaul, where the September 1994 eruptions occurred 10 years after the main seismic crisis.
If earthquakes or other events at Okataina suggest the possibility of an impending eruption, monitoring efforts by scientists from government departments and universities will be increased, with the aim of better assessment of the likelihood, size, location, and effects of any eruption. Scientific advice will be passed on to Civil Defence and local, regional, and national government agencies, who will advise the public in detail about the potential hazards and the public safety actions to be carried out. These actions may include evacuation of threatened areas, closure of roads and airports, and restrictions on entry into dangerous zones.
Figure 12: Surveying across the craters formed on Mt Tarawera in the 1886 AD eruption. Measurements are carried out at Tarawera and Rotomahana to detect any swelling of the ground surface after earthquake swarms in the vicinity.
Deformation of the ground surface above the earthquake locations will probably precede and would help identify an impending eruption, if the deformation occurs within previously surveyed networks, such as those installed at Tarawera-Rotomahana, or near lakes used as tilt-level indicators, so that it can be instrumentally measured (see Figure 12). Ground deformation occurring elsewhere may not be detectable until becoming visible to the naked eye, probably immediately prior to the eruption.
The short duration anticipated for precursory seismicity and ground deformation suggests that a basaltic eruption may not be usefully predicted. The eruption may occur before it could be confidently recognised as definitely impending.
In contrast, a rhyolite eruption is likely to be preceded by a long period (months or years) of increased shallow seismicity, with noticeable ground deformation occurring above the potential vent areas, changes in lake levels, and possibly increased heat flows from hot springs and fumarolic (steaming) areas. This is because rhyolitic eruptions are thought to come from large (10-100 km3?) viscous magma bodies stored at shallow depths (5-10 km?) in the earth’s crust. Magma intrusions rising from these large bodies will slowly fracture their way towards the surface.
Unlike basaltic eruptions, precursory indications of an impending rhyolitic eruption are likely to be recognised long before the event. However, while all large rhyolite eruptions are likely to be predicted, these predictions may include many false alarms. During the 1980s, active calderas in Italy, the United States and Papua New Gineau, went through periods of considerable unrest. Levels of seismic activity increased greatly, and vertical ground deformation (swelling) of 1m or larger was measured. These changes, although alarming and best explained by underground movement of magma, have not (as yet) led to eruptions.* It appears that calderas may undergo periods of considerable disturbance more frequently than actual eruptions may occur. There is no immediate one-to-one correlation between unrest and eruption. Thus, if a period of unrest occurs at Okataina, with greatly increased seismicity and ground deformation, it will indicate an increased probability, rather than a certainty, of impending eruption.
*Except at Rabaul, where the September 1994 eruptions occurred 10 years after the main seismic crisis.
If earthquakes or other events at Okataina suggest the possibility of an impending eruption, monitoring efforts by scientists from government departments and universities will be increased, with the aim of better assessment of the likelihood, size, location, and effects of any eruption. Scientific advice will be passed on to Civil Defence and local, regional, and national government agencies, who will advise the public in detail about the potential hazards and the public safety actions to be carried out. These actions may include evacuation of threatened areas, closure of roads and airports, and restrictions on entry into dangerous zones.
Figure 12: Surveying across the craters formed on Mt Tarawera in the 1886 AD eruption. Measurements are carried out at Tarawera and Rotomahana to detect any swelling of the ground surface after earthquake swarms in the vicinity.
What To Do In An Eruption At Okataina
Detailed instructions on actions to be taken during an impending eruption will be provided by Civil Defence. However, some general principles to follow if an eruption occurs without useful warning are set out here.
An eruption can be expected to block roads, cut water, electrical power, and telephone services, and threaten damage to buildings by build up of ash falling on flat roofs. Early evacuation of near-vent areas (within 5-20 km) is necessary and would be advised by radio; elsewhere residents will be advised to shelter indoors. If forced to move during an eruption, try to avoid areas immediately downwind of the volcano where ash fall will be thickest. Instead, travel across the wind direction if this is possible, watch for damage to roads and avoid panic driving in poor visibility. If staying indoors, it may be possible to remove ash from flat roofs during any breaks in ash fall; this will help reduce structural damage caused by ash build-up, which is particularly likely if the ash becomes wet by rainfall, which greatly increases loadings. In heavy ash falls breathing may become difficult due to the presence of fine dust and volcanic gases. Use filter masks, or substitute by breathing through layers of wet cloth. In areas close to active vents, if escape is impossible, shelter in the most substantial buildings and avoid windows facing the vents. These windows may be blown inwards by the shock waves produced by volcanic explosions. Residents on lake shores facing erupting vents should be aware of potential large waves and if possible move away from low lying shore areas to protection at higher levels.
An eruption can be expected to block roads, cut water, electrical power, and telephone services, and threaten damage to buildings by build up of ash falling on flat roofs. Early evacuation of near-vent areas (within 5-20 km) is necessary and would be advised by radio; elsewhere residents will be advised to shelter indoors. If forced to move during an eruption, try to avoid areas immediately downwind of the volcano where ash fall will be thickest. Instead, travel across the wind direction if this is possible, watch for damage to roads and avoid panic driving in poor visibility. If staying indoors, it may be possible to remove ash from flat roofs during any breaks in ash fall; this will help reduce structural damage caused by ash build-up, which is particularly likely if the ash becomes wet by rainfall, which greatly increases loadings. In heavy ash falls breathing may become difficult due to the presence of fine dust and volcanic gases. Use filter masks, or substitute by breathing through layers of wet cloth. In areas close to active vents, if escape is impossible, shelter in the most substantial buildings and avoid windows facing the vents. These windows may be blown inwards by the shock waves produced by volcanic explosions. Residents on lake shores facing erupting vents should be aware of potential large waves and if possible move away from low lying shore areas to protection at higher levels.
ADDITIONAL INFORMATION
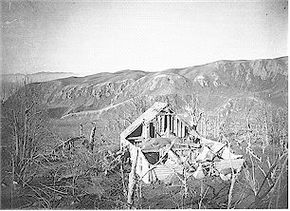
Collapse of the Te Mu Parsonage building at Te Wairoa caused by accumulation of volcanic ash during the 1886 eruption. Photo by C. Spencer.
More detailed information about volcanic history and processes at Okataina, and about volcanic activity in general, is contained in the following publications:
Blong, R.J. 1984: Volcanic hazards: A sourcebook on the effects of eruptions. Academic Press Australia. North Ryde, New South Wales.
Keam, R.F. 1988: Tarawera: the volcanic eruption of 10 June 1886. R.F. Keam – Auckland, New Zealand. 472 pages.
Nairn, I.A. 1989: Sheet V16AC-Mount Tarawera. Geological Map of New Zealand 1:50,000 Map (1 sheet) and notes.Wellington, New Zealand. Department of Scientific and Industrial Research.
Scott, B.J. and Nairn, I.A. 1998: Volcanic hazard map of Okataina Volcanic Centre. Environment Bay of Plenty resource planning publication 97/4.
More detailed information about volcanic history and processes at Okataina, and about volcanic activity in general, is contained in the following publications:
Blong, R.J. 1984: Volcanic hazards: A sourcebook on the effects of eruptions. Academic Press Australia. North Ryde, New South Wales.
Keam, R.F. 1988: Tarawera: the volcanic eruption of 10 June 1886. R.F. Keam – Auckland, New Zealand. 472 pages.
Nairn, I.A. 1989: Sheet V16AC-Mount Tarawera. Geological Map of New Zealand 1:50,000 Map (1 sheet) and notes.Wellington, New Zealand. Department of Scientific and Industrial Research.
Scott, B.J. and Nairn, I.A. 1998: Volcanic hazard map of Okataina Volcanic Centre. Environment Bay of Plenty resource planning publication 97/4.